泰达国际心血管病医院 郑 刚
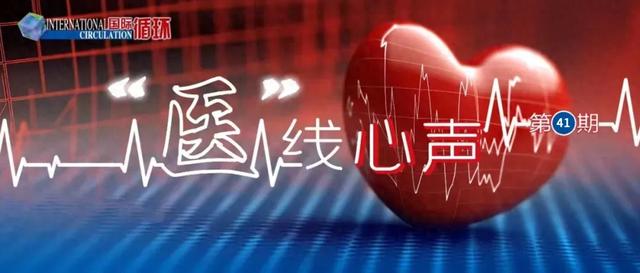
尽管目前临床上有很好的药物治疗和血运重建方法,但动脉粥样硬化性心血管疾病(ASCVD)仍然是发病率和死亡率的主要原因。在开创性的4S试验之后,临床上广泛使用他汀类药物降低低密度脂蛋白胆固醇(LDL-C)预防复发性心肌梗死(MI)、卒中和心血管死亡[1]。他汀类药物试验显示,患者广泛受益于他汀类药物,包括那些没有明显血管疾病或高脂血症证据的患者[2]。在接受最佳治疗的有临床血管疾病患者中,相当大比例(>20%甚至>30%)的患者在10年期间仍有复发事件(例如MI、中风或血管性死亡)的风险[3]。
一个多世纪前,Rudolf Virchow提出动脉粥样硬化是由胆固醇诱导的慢性炎症状态[4]。除了来自降低LDL-C的研究数据之外,PESA研究发现,在没有常规 ASCVD危险因素(例如吸烟和高血压)的健康个体中,LDL-C与动脉粥样硬化负荷(通过亚临床动脉粥样硬化评估)之间存在独立和直接的联系[5]。事实上,随着时间的推移,维持低LDL-C会减少动脉壁内的LDL颗粒数量,减缓动脉粥样硬化的进展,延迟成熟动脉粥样硬化斑块发展的年龄,并大大降低ASCVD事件的终生风险[6]。
考虑到许多急性心肌梗死(AMI)患者的LDL-C不高,但有炎症增加的特征,因此提出了炎症假说[7],即慢性和急性形式的炎症可能是尚未被充分认识的心血管危险因素之一[8]。来自概念验证CANTOS试验的数据已经清楚地确定炎症是导致冠状动脉事件的关键生物学过程之一[9]。同样,在COLCOT研究中,与安慰剂相比,低剂量秋水仙素有效预防了近期MI患者的主要不良心血管事件(MACE)的发生[10]。因此,美国食品和药物管理局(FDA)批准使用低剂量秋水仙碱治疗已确诊的ASCVD患者。此外,欧洲心脏病学会指南建议考虑秋水仙碱作为预防急性冠状动脉综合征(ACS)患者复发性心血管事件的治疗标准[11]。COLCOT研究表明,在ACS患者中,秋水仙素可稳定斑块,表现为最小纤维帽厚度增加,脂质弧减少,巨噬细胞浸润减少[12]。相反,COCOMO-ACS试验发现,光学相干断层扫描(OCT)没有检测到秋水仙素对最小纤维帽厚度、非罪犯病变成像片段或富含脂质斑块最大脂质弧的显著影响[13]。因此,鉴定可用于临床实践的炎症生物标志物(例如白细胞介素-6[IL6]或IL-1β)有望靶向正确的患者以减少ASCVD[14]。
除此之外,在接受强化降脂治疗的患者中,高敏感性C反应蛋白(hs-CRP) 比单独使用LDL更能预测未来的心血管事件[15-16]。hs-CRP 水平升高与复发性心血管事件和死亡风险增加有关,无论涉及的血管范围如何(即:冠状动脉疾病、脑血管疾病、周围动脉阻塞性疾病和腹主动脉瘤)[17]。前瞻性研究还表明,术前 hs-CRP水平升高与术后早期再狭窄的风险增加有关[18]。值得注意的是,在1069 名接受动脉内膜切除术的患者中,hs-CRP水平≥2 mg/L 的患者表现出促炎症和不良斑块表型,拥有属性升高斑块细胞因子水平的IL-6和IL-8[19]。
由于慢性炎症标志着脂质介导的动脉粥样硬化形成,因此在家族性高胆固醇血症(FH)患者中不能忽视炎症状态的存在[20]。FH是一种代谢疾病,由于血液LDL颗粒的肝脏摄取受损,引发代偿性胆固醇合成增加,并最终导致高血浆LDL水平。低密度脂蛋白受体(LDLR)依赖途径对LDL的摄取受到LDLR、载脂蛋白 B(ApoB)和前蛋白转化酶枯草杆菌蛋白酶9型(PCSK9)基因的遗传变异的影响[21]。FH作为常染色体显性遗传疾病,可以区分为单等位基因或双等位基因。第一种情况称为杂合性FH(HeFH),是最常见的单基因疾病,估计在200~350名受试者中有1名患病[22-25]。第二种情况通常称为纯合性FH(HoFH),估计患病率为1/160~300 000[21,25,26]。HoFH也可以由LDLR相关蛋白1(LDLRAP1)中的变体引起,LDLRAP1作为常染色体隐性疾病,这种形式的FH<1% ,是由LDLRAP1的双等位基因功能丧失变异引起的[27-28]。
自婴儿时期以来一直暴露于增加的LDL浓度,与早产儿冠状动脉疾病的风险增加有关。然而,后者的表达存在相当大的差异性[29]。一方面,高达45%的HeFH 受试者没有表现出任何ASCVD,并且一些HoFH 患者也被报道对动脉粥样硬化具有弹性[30,31]。相反,尽管具有相同的遗传和环境背景,一些患者的疾病进程更迅速,尽管使用他汀类药物治疗,但是仍进展为早期心血管疾病[32]。然而,早期诊断和治疗对于减轻过度的心血管风险是必不可少的[33]。此外,炎症是自身免疫性疾病患者心血管风险的主要因素[34]。基于这一背景,本文概述与LDL-C水平升高相关的主要炎症分子途径,包括与FH相关的途径,并描述脂质降低方法在改善炎症和脂质负担中的差异。
1、动脉粥样硬化和炎症:分子概述
斑块破裂的关键因素包括炎症范围[35]、脂质核心大小、纤维帽厚度、平滑肌细胞凋亡、蛋白水解酶和斑块钙化之间的平衡[36]。免疫细胞组成的变化[37],包括巨噬细胞、树突状细胞、T细胞、B细胞、肥大细胞和嗜中性粒细胞,以及细胞因子和趋化因子释放的改变,破坏了斑块部位炎症和抗炎机制之间的平衡[38]。动脉粥样硬化形成的最初步骤涉及ApoB在含脂蛋白的内膜下聚集。对这些残留脂蛋白的局部生物学反应,包括以巨噬细胞和T细胞为主的慢性和不适应性炎症反应,有助于随后的病变形成,动脉粥样硬化的炎症性质由随后的内皮功能障碍证实[39],导致与疾病进展相关的内皮细胞促粘附和促血栓形成[40]。剪切应力在早期动脉粥样硬化中的作用主要通过刺激壁内合成分子(例如蛋白多糖)来介导,其促进脂蛋白的保留[39]。鉴于其暴露于有害刺激,内皮细胞的特殊敏感性是至关重要的。因此,它是一个广泛过程的一部分,涉及几种免疫和炎症介质,在胆固醇升高的情况下会出现更严重的后果。
在动脉粥样硬化形成的背景下,脂筏功能失调的维持起着重要作用。脂筏是由于胆固醇和鞘脂之间的分子相互作用而形成的[41]。在膜水平上,这些脂筏通过聚集受体产生敏感区域,这影响白细胞的滚动并粘附到内皮细胞上。激活后,脂筏内驻留和募集的蛋白质启动导致炎症的信号级联[42]。此外,脂筏已被认为在细胞外囊泡(EV)的产生和组成中起作用[43]。这些是小磷脂双层分界颗粒的异质群体,没有细胞核,从大多数生物体液中的所有细胞类型释放[44]。虽然一度被认为是一种消除细胞废物的手段,EV现在已知在各种生理和病理过程中发挥关键作用[45]。它们被认为是细胞间通信的关键参与者,因为它们能够运输蛋白质、脂质和核酸。EV涉及多种心血管疾病,包括动脉粥样硬化[46-47]、心力衰竭、心肌病变[48]和心室颤动[49]。对脂筏作用的进一步了解在调节血管炎症的过程中已经变得特别重要,并且在预防和治疗ASCVD方面具有潜力[50]。
脂蛋白和炎症之间的进一步联系源于长期或过度暴露于氧化磷脂。氧化磷脂通常由促动脉粥样硬化脂蛋白(a)[Lp(a)]颗粒携带。氧化磷脂作用于各种血管细胞上的特定受体,包括分化簇(CD)36和其他清道夫受体CD14、Toll样受体和氧化型低密度脂蛋白(oxLDL)受体1(也称为 LOX1),触发促炎级联反应[51]。与Toll样受体一起,清道夫受体在内化修饰的LDL白颗粒(通常是oxLDL)中具有基础作用。在新生斑块内,斑块巨噬细胞通过清道夫受体内化氧化脂质,并分化为泡沫细胞[52]。
巨噬细胞通常细分为两个亚组:M1巨噬细胞(促炎)和M2巨噬细胞(抗炎)。尽管M1的形成是由促炎细胞因子或产生炎性细胞因子(IL-1β、IL-6、IL-12、IL-23)和肿瘤坏死因子-α(TNF-α)的病原体诱导的,但是M2是由细胞因子IL-4和IL-13诱导的,并产生 IL-10,后者具有抗炎特性。在早期动脉粥样硬化病变中,M1存在,随着斑块向更复杂的炎症病变发展,M1的比例增加。相反,在动脉粥样硬化消退期间,M1和M2之间的平衡发生了变化,M2变得更加突出[53]。M1的活化主要依赖于无氧糖酵解,导致显著的代谢重新连接,这拥有属性增加了葡萄糖摄取和增强了有氧糖酵解。这种新陈代谢的变化与氧化磷酸化通过三羧酸循环受损相一致。相比之下,M2表现出大幅增加的脂肪酸氧化和氧化磷酸化,这被认为是有助于抗炎反应[54]。
NLRP3组装胞质先天性免疫复合物,其激活半胱氨酸蛋白酶半胱天冬酶 -1,其依次切割细胞凋亡D(gasdermin D)以诱导细胞焦亡(pyroptosis),裂解细胞死亡的调节模式[56]。这些步骤有利于斑块巨噬细胞产生更高数量的IL-1β[57],从而扩大炎症相关的转导途径[58]。来自经历细胞内结晶饱和脂肪酸的促炎信号也可以通过Toll样受体,特别是亚型4,激活炎症级联反应[59]。在这种情况下,Yalcinkaya 等[60]认为胆固醇在质膜胆固醇和内质网之间的转运触发了炎症小体的激活。淋巴细胞T辅助细胞1(Th1)具有促动脉粥样硬化作用,T调节细胞(Treg)具有抗动脉粥样硬化作用,尽管后者的功能可能在特定条件下发生变化。免疫适应细胞具有促炎(Th1或Th17)淋巴细胞和抗炎(Treg)淋巴细胞的特性。Th1在动脉粥样硬化斑块中大量存在,并且通过有利于促炎细胞因子的释放激活斑块中的巨噬细胞、淋巴细胞和其他细胞,从而提高炎症反应。
这种细胞因子对动脉粥样硬化有混合作用,可能促进炎症和增加纤维化[62]。具有抗炎特性的Treg细胞在动脉粥样硬化过程中可能失去这些特征。ASCVD患者Tregs中的胆固醇积累可能会增强向促动脉粥样硬化T细胞亚群的分化[63]。与稳定斑块相比,易损斑块中检测到Tregs数量减少[64]。此外,作为T细胞亚群的组织驻留,记忆T细胞(TRM)位于先前的抗原识别部位,提供防止再次发生的保护[65]。尽管TRM在动脉粥样硬化病变中是一个小群体,但与病变稳定性增加有关[66]。
B细胞在动脉粥样硬化中的作用是复杂和多方面的。虽然一些证据表明B细胞在影响动脉粥样硬化斑块的进展中起重要作用,但其他研究已经显示B细胞群对斑块形成的竞争作用[67]。在体液反应的情况下,反映半乳糖化和唾液酸化模式的IgG的 N-糖基化始终与ASCVD事件的复发有关。IgG糖基化模式的改变可以调节IgG受体结合,直接影响炎症级联反应[68]。
在动脉粥样硬化发展过程中,活化的中性粒细胞颗粒促进单核细胞的募集和分泌活性氧和蛋白酶。这个过程使内皮细胞层失调,使LDL渗出。随着动脉粥样硬化的进展,中性粒细胞也分泌髓过氧化物酶和oxLDL颗粒,进一步形成泡沫细胞。在晚期动脉粥样硬化病变中,嗜中性粒细胞可以通过分泌嗜中性粒细胞胞外陷阱(NETs)破坏斑块的稳定性,这种陷阱会穿孔和裂解血管平滑肌细胞,导致纤维帽变薄和易破裂斑块的形成[69]。观察数据和遗传数据都支持血液中性粒细胞计数升高 ASCVD的一个因果危险因素的假设[70]。在高龄骨髓相关的变化通常有两个拥有属性:①NETosis,这是一种主要的细菌杀伤机制,但也是无菌炎症如动脉疾病的主要机制[71],②嗜中性粒细胞的表观遗传学变化,可能导致与低度炎症相关的功能障碍,改变免疫反应和促进炎症反应[72]。NETs和NETosis增加了动脉粥样硬化病变中性粒细胞浸润的解剖学标志。骨髓最初被认为不是动脉内膜中炎性细胞募集(特别是单核细胞)增强的原因[74] ,但最近在外周动脉疾病患者中遇到了循环造血祖细胞的明确减少[75]。
突变造血细胞的克隆扩增,称为克隆造血,在老年人中很常见,并导致 ASCVD 风险升高[76]。克隆性造血减少了与动脉粥样硬化风险升高相关的表观遗传修饰酶 ten-November易位 2(TET2)[77]。这种表观遗传修饰物是动脉粥样硬化炎症模式中的有效中介,并在巨噬细胞的表观遗传重塑中被鉴定。通过DNA甲基化或组蛋白修饰(例如甲基化、乙酰化和乳酸化),TET2 通过基因表达的转录重编程和细胞内代谢重新连接来确定或切换巨噬细胞极化,从而代表动脉疾病抗炎治疗的潜在目标[78]。不确定潜能克隆性造血的直接临床评价可能为提高大量心脏病患者特异性抗炎治疗的可行性提供一种实用的方法[79]。克隆性造血,特别是在TET2 缺陷细胞中,可显著增加斑块大小和NLRP3炎性体介导的IL-1β分泌。CANTOS研究的一项探索性分析显示,TET2体细胞变异导致不确定性造血的患者在使用卡那单抗(canakinumab)时降低了MACE的风险。DNA甲基转移酶3A(DNMT3A)的基因突变尤其令人感兴趣[80]。
DNMT3A催化与基因沉默相关的胞嘧啶甲基化,而TET2编码甲基胞嘧啶双加氧酶启动导致胞嘧啶去甲基化的一系列反应[81]。DNMT3A的缺失增强了体内巨噬细胞的炎症反应,并在体内产生了一个独特的外膜巨噬细胞群,它将巨噬细胞特征与炎性细胞因子标记结合起来[82]。另一个有趣的机制连接胆固醇和炎症涉及半乳糖凝集素-9(gal9),成员的 β-半乳糖苷结合蛋白家族表达的内皮在炎症环境。在高脂饮食后,apoE-/-、Gal9-/- 小鼠与ApoE-/- 同窝小鼠相比,主动脉斑块负荷显著降低[83]。
反向胆固醇转运(rCT)是一种高度抗动脉粥样硬化的过程,负责过量胆固醇向载脂蛋白A1的流出[84]。炎症可以阻碍rCT途径的几个步骤,特别是胆固醇通过肝脏流向胆汁和粪便[85]。有缺陷的胆固醇流出促进骨髓和脾脏中的单核细胞和嗜中性粒细胞的产生,涉及造血干细胞和骨髓祖细胞,造血干细胞和髓外造血的动员[86]。
专家简介
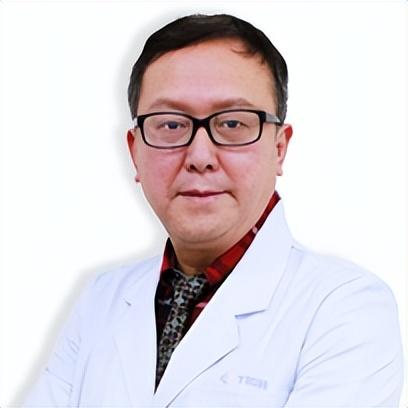
郑刚 教授
现任泰达国际心血管病医院特聘专家,济兴医院副院长
中国高血压联盟理事,中国心力衰竭学会委员,中国老年医学会高血压分会天津工作组副组长,中国医疗保健国际交流促进会高血压分会委员
天津医学会心血管病专业委员会委员,天津医学会老年病专业委员会常委,天津市医师协会高血压专业委员会常委,天津市医师协会老年病专业委员会委员,天津市医师协会心力衰竭专业委员,天津市医师协会心血管内科医师分会双心专业委员会委员,天津市心脏学会理事,天津市心律学会第一届委员会委员,天津市房颤中心联盟常委,天津市医药学专家协会第一届心血管专业委员会委员,天津市药理学会临床心血管药理专业委员会常委,天津市中西医结合学会心血管疾病专业委员会常委
《中华临床医师杂志(电子版)》特邀审稿专家,《中华诊断学电子杂志》《心血管外科杂志(电子版)》审稿专家,《华夏医学》副主编,《中国心血管杂志》常务编委,《中国心血管病研究》杂志第四届编委,《中华老年心脑血管病杂志》《世界临床药物》《医学综述》《中国医药导报》《中国现代医生》编委
本人在专业期刊和心血管网发表文章979篇,其中第一作者790篇,参加著书11部。获天津市2005年度“五一劳动奖章和奖状”和“天津市卫生行业第二届人民满意的好医生”称号
参考文献
(上下滑动可查看)
1. Randomised trial of cholesterol lowering in 4444 patients with coronary heart disease: the Scandinavian simvastatin survival study (4S). Lancet. 1994;344(8934):1383-1389.
2. Cholesterol Treatment Trialists' (CTT) Collaboration, Fulcher J, O'Connell R, et al. Efficacy and safety of LDL-lowering
therapy among men and women: meta-analysis of individual data from 174,000 participants in 27 randomised trials. Lancet.
2015;385(9976):1397-1405.
3. Kaasenbrood L, Boekholdt SM, van der Graaf Y, et al. Distribution of estimated 10-year risk of recurrent vascular
events and residual risk in a secondary prevention population. Circulation. 2016;134(19):1419-1429.
4. Lawler PR, Bhatt DL, Godoy LC, et al. Targeting cardiovascular inflammation: next steps in clinical translation. Eur Heart J.
2021;42(1):113-131.
5. Fernandez-Friera L, Fuster V, Lopez-Melgar B, et al. Normal LDL-Cholesterol Levels are associated with subclinical ath
erosclerosis in the absence of risk factors. J Am Coll Cardiol. 2017;70(24):2979-2991.
6. Ference BA, Braunwald E, Catapano AL. The LDL cumulative exposure hypothesis: evidence and practical applications. Nat
Rev Cardiol. 2024;21:701-716.
7. Libby P, Smith R, Rubin EJ, Glassberg MK, Farkouh ME, Rosenson RS. Inflammation unites diverse acute and chronic
diseases. Eur J Clin Investig. 2024;e14280. doi:10.1111/ eci.14280
8. Matter MA, Paneni F, Libby P, et al. Inflammation in acute myocardial infarction: the good, the bad and the ugly. Eur Heart
J. 2024;45(2):89-103.
9. Ridker PM, Everett BM, Thuren T, et al. Antiinflammatory therapy with Canakinumab for atherosclerotic disease. N Engl
J Med. 2017;377(12):1119-1131.
10. Tardif JC, Kouz S, Waters DD, et al. Efficacy and safety of low-dose colchicine after myocardial infarction. N Engl J Med.
2019;381(26):2497-2505.
11. Byrne RA, Rossello X, Coughlan JJ, et al. 2023 ESC guidelines for the management of acute coronary syndromes. Eur Heart J Acute Cardiovasc Care. 2024;13(1):55-161.
12. Yu M, Yang Y, Dong SL, et al. Effect of colchicine on coronary plaque stability in acute coronary syndrome as assessed by optical coherence tomography: the COLOCT randomized clinical trial. Circulation. 2024;150:981-993.
13. Psaltis PJ, Nguyen MT, Singh K, et al. Optical coherence tomography assessment of the impact of colchicine on non-culprit coronary plaque composition after myocardial infarction. Cardiovasc Res. 2024;cvae191. doi:10.1093/cvr/cvae191
14. Held C, White HD, Stewart RAH, et al. Inflammatory biomarkers interleukin-6 and C-reactive protein and outcomes in stable coronary heart disease: experiences from the STABILITY (stabilization of atherosclerotic plaque by initiation of darapladib therapy). Trial J Am Heart Assoc. 2017;6(10):e005077.
15. Ridker PM, Bhatt DL, Pradhan AD, et al. Inflammation and cholesterol as predictors of cardiovascular events among pa
tients receiving statin therapy: a collaborative analysis of three randomised trials. Lancet. 2023;401(10384):1293-1301.
16. Ridker PM, Lei L, Louie MJ, et al. Inflammation and Cholesterol as predictors of cardiovascular events among 13 970 contemporary high-risk patients with statin intolerance. Circulation. 2024;149(1):28-35.
17. Burger PM, Pradhan AD, Dorresteijn JAN, et al. C-reactive protein and risk of cardiovascular events and mortality in patients with various cardiovascular disease locations. Am J Cardiol. 2023;197:13-23.
18. Zhou F, Hua Y, Ji X, Jia L. A systemic review into carotid plaque features as predictors of restenosis after carotid endarterectomy. J Vasc Surg. 2021;73(6):2179-2188.
19. Kraaijenhof JM. Plasma C-reactive protein is associated with a pro-inflammatory and adverse plaque phenotype.
Atherosclerosis. 2024;396:118532.
20. Montecucco F, Carbone F, Liberale L, Sahebkar A. Challenges in reducing atherosclerotic inflammation in patients
with familial hypercholesterolemia. Eur J Prev Cardiol. 2020;27(19):2099-2101.
21. Cuchel M, Raal FJ, Hegele RA, et al. 2023 update on European atherosclerosis society consensus statement on homozygous familial Hypercholesterolaemia: new treatments and clinical guidance. Eur Heart J. 2023;44(25):2277-2291.
22. Hu P, Dharmayat KI, Stevens CAT, et al. Prevalence of familial hypercholesterolemia among the general population and pa
tients with atherosclerotic cardiovascular disease: a systematic review and meta-analysis. Circulation. 2020;141(22):1742-1759.
23. Beheshti SO, Madsen CM, Varbo A, Nordestgaard BG. Worldwide prevalence of familial hypercholesterolemia:
meta-analyses of 11 million subjects. J Am Coll Cardiol. 2020;75(20):2553-2566.
24. Collaboration EASFHS. Global perspective of familial hypercholesterolaemia: a cross-sectional study from the EAS familial
Hypercholesterolaemia studies collaboration (FHSC). Lancet. 2021;398(10312):1713-1725.
25. Defesche JC, Gidding SS, Harada-Shiba M, Hegele RA, Santos RD, Wierzbicki AS. Familial hypercholesterolaemia. Nat Rev
Dis Prim. 2017;3:17093.
26. Hegele RA, Boren J, Ginsberg HN, et al. Rare dyslipidaemias, from phenotype to genotype to management: a European atherosclerosis society task force consensus statement. Lancet Diabetes Endocrinols. 2020;8(1):50-67.
27. D'Erasmo L, Di Costanzo A, Arca M. Autosomal recessive hypercholesterolemia: update for 2020. Curr Opin Lipidol.
2020;31(2):56-61.
28. Chaudhry A, Trinder M, Vesely K, et al. Genetic identification of homozygous familial hypercholesterolemia by long-read
sequencing among patients with clinically diagnosed heterozygous familial hypercholesterolemia. Circ Genom Precis Med.
2023;16(2):e003887.
29. Tada H, Kaneko H, Suzuki Y, et al. Familial hypercholesterolemia is related to cardiovascular disease, heart failure and atrial fibrillation. Results from a population-based study. Eur J Clin Investig. 2024;54(2):e14119.
30. Mszar R, Grandhi GR, Valero-Elizondo J, et al. Absence of coronary artery calcification in middle-aged familial hypercho
lesterolemia patients without atherosclerotic cardiovascular disease. JACC Cardiovasc Imaging. 2020;13(4):1090-1092.
31. Nasir K, Mszar R, Cainzos-Achirica M, et al. Age- and sex-based heterogeneity in coronary artery plaque presence and burden in familial hypercholesterolemia: a multi-national study. Am J Prev Cardiol. 2024;17:100611.
32. Brautbar A, Leary E, Rasmussen K, Wilson DP, Steiner RD, Virani S. Genetics of familial hypercholesterolemia. Curr
Atheroscler Rep. 2015;17(4):491.
33. Mulder J, Tromp TR, Al-Khnifsawi M, et al. Sex differences in diagnosis, Treatment, and cardiovascular outcomes in ho
mozygous familial hypercholesterolemia. JAMA Cardiol. 2024;9(4):313-322.
34. Porsch F, Binder CJ. Autoimmune diseases and atherosclerotic cardiovascular disease. Nat Rev Cardiol. 2024. doi:10.1038/
s41569-024-01045-7
35. Attiq A, Afzal S, Ahmad W, Kandeel M. Hegemony of inflammation in atherosclerosis and coronary artery disease. Eur J
Pharmacol. 2024;966:176338.
36. Stefanadis C, Antoniou CK, Tsiachris D, Pietri P. Coronary atherosclerotic vulnerable plaque: current perspectives. J Am
Heart Assoc. 2017;6(3):e005543.
37. Fleetwood AJ, Noonan J, La Gruta N, Kallies A, Murphy AJ. Immunometabolism in atherosclerotic disorders. Nat
Cardiovasc Res. 2024;3(6):637-650.
38. Certo M, Rahimzadeh M, Mauro C. Immunometabolism in atherosclerosis: a new understanding of an old disease. Trends
Biochem Sci. 2024;49:791-803.
39. Tabas I, Williams KJ, Boren J. Subendothelial lipoprotein retention as the initiating process in atherosclerosis: update and
therapeutic implications. Circulation. 2007;116(16):1832-1844.
40. Gimbrone MA Jr, Garcia-Cardena G. Endothelial cell dysfunction and the pathobiology of atherosclerosis. Circ Res.
2016;118(4):620-636.
41. Sorci-Thomas MG, Thomas MJ. Microdomains, inflammation, and atherosclerosis. Circ Res. 2016;118(4):679-691.
42. Li S, Navia-Pelaez JM, Choi SH, Miller YI. Macrophage inflammarafts in atherosclerosis. Curr Opin Lipidol.
2023;34(5):189-195.
43. Ouweneel AB, Thomas MJ, Sorci-Thomas MG. The ins and outs of lipid rafts: functions in intracellular cholesterol homeostasis, microparticles, and cell membranes: thematic review series: biology of lipid rafts. J Lipid Res. 2020;61(5):676-686.
44. van Niel G, Carter DRF, Clayton A, Lambert DW, Raposo G, Vader P. Challenges and directions in studying cell-cell com
munication by extracellular vesicles. Nat Rev Mol Cell Biol. 2022;23(5):369-382.
45. Rizzuto AS, Gelpi G, Mangini A, Carugo S, Ruscica M, Macchi C. Exploring the role of epicardial adipose-tissue-derived
extracellular vesicles in cardiovascular diseases. iScience. 2024;27(4):109359.
46. Camera M, Brambilla M, Canzano P, et al. Association of Microvesicles with Graft Patency in patients undergoing CABG
surgery. J Am Coll Cardiol. 2020;75(22):2819-2832.
47. Greco MF, Rizzuto AS, Zara M, et al. PCSK9 confers inflammatory properties to extracellular vesicles released by vascular
smooth muscle cells. Int J Mol Sci. 2022;23(21):13065.
48. Rizzuto AS, Faggiano A, Macchi C, Carugo S, Perrino C, Ruscica M. Extracellular vesicles in cardiomyopathies: a narra
tive review. Heliyon. 2024;10(1):e23765.
49. Shaihov-Teper O, Ram E, Ballan N, et al. Extracellular vesicles from epicardial fat facilitate atrial fibrillation. Circulation.
2021;143(25):2475-2493.
50. Sviridov D, Mukhamedova N, Miller YI. Lipid rafts as a therapeutic target. J Lipid Res. 2020;61(5):687-695.
51. Tsimikas S, Witztum JL. Oxidized phospholipids in cardiovascular disease. Nat Rev Cardiol. 2024;21(3):170-191.
52. Kzhyshkowska J, Neyen C, Gordon S. Role of macrophage scavenger receptors in atherosclerosis. Immunobiology.
2012;217(5):492-502.
53. Feig JE, Parathath S, Rong JX, et al. Reversal of hyperlipidemia with a genetic switch favorably affects the content and
inflammatory state of macrophages in atherosclerotic plaques. Circulation. 2011;123(9):989-998.
54. Koelwyn GJ, Corr EM, Erbay E, Moore KJ. Regulation of macrophage immunometabolism in atherosclerosis. Nat Immunol.
2018;19(6):526-537.
55. Duewell P, Kono H, Rayner KJ, et al. NLRP3 inflammasomes are required for atherogenesis and activated by cholesterol crystals. Nature. 2010;464(7293):1357-1361.
56. Vande Walle L, Lamkanfi M. Drugging the NLRP3 inflammasome: from signalling mechanisms to therapeutic targets.
Nat Rev Drug Discov. 2024;23(1):43-66.
57. Jin Y, Fu J. Novel insights into the NLRP 3 Inflammasome in atherosclerosis. J Am Heart Assoc. 2019;8(12):e012219.
58. Karasawa T, Kawashima A, Usui-Kawanishi F, et al. Saturated fatty acids undergo intracellular crystallization and activate the NLRP3 Inflammasome in macrophages. Arterioscler Thromb Vasc Biol. 2018;38(4):744-756.
59. Yalcinkaya M, Liu W, Xiao T, et al. Cholesterol trafficking to the ER leads to the activation of CaMKII/JNK/NLRP3 and promotes atherosclerosis. J Lipid Res. 2024;65(4):100534.
60. Gistera A, Hansson GK. The immunology of atherosclerosis. Nat Rev Nephrol. 2017;13(6):368-380.
61. Bellini R, Bonacina F, Norata GD. Crosstalk between dendriticcells and T lymphocytes during atherogenesis: focus on antigen presentation and break of tolerance. Front Cardiovasc Med. 2022;9:934314.
62. Libby P, Loscalzo J, Ridker PM, et al. Inflammation, immunity, and infection in atherothrombosis: JACC review topic of the
week. J Am Coll Cardiol. 2018;72(17):2071-2081.
63. Bazioti V, Halmos B, Westerterp M. T-cell Cholesterol accumulation, aging, and atherosclerosis. Curr Atheroscler Rep.
2023;25(9):527-534.
64. Dietel B, Cicha I, Voskens CJ, Verhoeven E, Achenbach S, Garlichs CD. Decreased numbers of regulatory T cells are as
sociated with human atherosclerotic lesion vulnerability and inversely correlate with infiltrated mature dendritic cells.
Atherosclerosis. 2013;230(1):92-99.
65. Farber DL, Yudanin NA, Restifo NP. Human memory T cells: generation, compartmentalization and homeostasis. Nat Rev
Immunol. 2014;14(1):24-35.
66. de Jong MJM, Depuydt MAC, Schaftenaar FH, et al. Resident memory T cells in the atherosclerotic lesion associate with
reduced macrophage content and increased lesion stability. Arterioscler Thromb Vasc Biol. 2024;44(6):1318-1329.
67. Obare LM, Bonami RH, Doran AC, Wanjalla CN. B cells and atherosclerosis: a HIV perspective. J Cell Physiol.
2024;239(6):e31270.
68. Hoshi RA, Plavsa B, Liu Y, et al. N-glycosylation profiles of immunoglobulin G and future cardiovascular events. Circ Res.
2024;134(5):e3-e14.
69. Sreejit G, Johnson J, Jaggers RM, et al. Neutrophils in cardiovascular disease: warmongers, peacemakers, or both? Cardiovasc Res. 2022;118(12):2596-2609.
70. Luo J, Thomassen JQ, Nordestgaard BG, Tybjaerg-Hansen A, Frikke-Schmidt R. Neutrophil counts and cardiovascular dis
ease. Eur Heart J. 2023;44(47):4953-4964.
71. Jorch SK, Kubes P. An emerging role for neutrophil extracellular traps in noninfectious disease. Nat Med. 2017;23(3):
279-287.
72. Ecker S, Chen L, Pancaldi V, et al. Genome-wide analysis of differential transcriptional and epigenetic variability across
human immune cell types. Genome Biol. 2017;18(1):18.
73. Warnatsch A, Ioannou M, Wang Q, Papayannopoulos V. Inflammation. Neutrophil extracellular traps license macro
phages for cytokine production in atherosclerosis. Science. 2015;349(6245):316-320.
74. Massa M, Rosti V, Ferrario M, et al. Increased circulating hematopoietic and endothelial progenitor cells in the early phase of acute myocardial infarction. Blood. 2005;105(1):199-206.
75. Hayek SS, MacNamara J, Tahhan AS, et al. Circulating progenitor cells identify peripheral arterial disease in patients with coronary artery disease. Circ Res. 2016;119(4):564-571.
76. Petrone G, Turker I, Natarajan P, Bolton KL. Clinical and therapeutic implications of clonal hematopoiesis. Annu Rev
Genomics Hum Genet. 2024;25(1):329-351.
77. Jaiswal S, Fontanillas P, Flannick J, et al. Age-related clonal hematopoiesis associated with adverse outcomes. N Engl J Med. 2014;371(26):2488-2498.
78. Yang H, Sun Y, Li Q, Jin F, Dai Y. Diverse epigenetic regulations of macrophages in atherosclerosis. Front Cardiovasc Med.
2022;9:868788.
79. Sciatti E, D'Elia E, Gori M, et al. Clonal hematopoiesis of indeterminate potential: implications for the cardiologists. J
Cardiovasc Med (Hagerstown). 2024;25(1):1-12.
80. Fuster JJ, MacLauchlan S, Zuriaga MA, et al. Clonal hematopoiesis associated with TET2 deficiency accelerates atherosclerosis development in mice. Science. 2017;355(6327):842-847.
81. Svensson EC, Madar A, Campbell CD, et al. TET2-driven clonal hematopoiesis and response to canakinumab: an exploratory analysis of the CANTOS randomized clinical trial. JAMA Cardiol. 2022;7(5):521-528.
82. Rauch PJ, Gopakumar J, Silver AJ, et al. Loss-of-function mutations in Dnmt3a and Tet2 lead to accelerated atherosclerosis
and concordant macrophage phenotypes. Nat Cardiovasc Res. 2023;2(9):805-818.
83. Krautter F, Hussain MT, Zhi Z, et al. Galectin-9: a novel promoter of atherosclerosis progression. Atherosclerosis.
2022;363:57-68.
84. Adorni MP, Zimetti F, Cangiano B, et al. High-density lipoprotein function is reduced in patients affected by genetic or idiopathic hypogonadism. J Clin Endocrinol Metab. 2019;104(8):3097-3107.
85. McGillicuddy FC, de la Llera MM, Hinkle CC, et al. Inflammation impairs reverse cholesterol transport in vivo.
Circulation. 2009;119(8):1135-1145.
86. Tall AR, Yvan-Charvet L. Cholesterol, inflammation and innateimmunity. Nat Rev Immunol. 2015;15(2):104-116.